Orthogonal Nanometer-Micrometer Roughness Gradients
While contrary effects of roughness feature-size have been observed on osteoblasts (see Micro-Scale Morphological Gradients and Nano-Scale Morphological Gradients), an orthogonal nanometer-micrometer roughness gradients was used to study the effect of roughness feature-size on osteoblasts (see Fig. 1).
Method
In this technique, a micrometer-scale morphological gradient was prepared as described previously. Ceramic replicates of those samples were then created by taking a first imprint of the roughness gradient in polyvinylsiloxane and filling up this mould with an alumina slurry. The dried green-body was then recovered from the mould and sintered. A thin layer of SiO2 was the deposited to smoothen the surface at the grain asperities and to be able to generate in the orthogonal direction a nanoparticle gradient as described in the Nano-Scale Morphological Gradients section, under ultrasonication to ensure nanoparticle adsorption in the deep grooves of the micrometer-feature gradient (see Fig. 2).
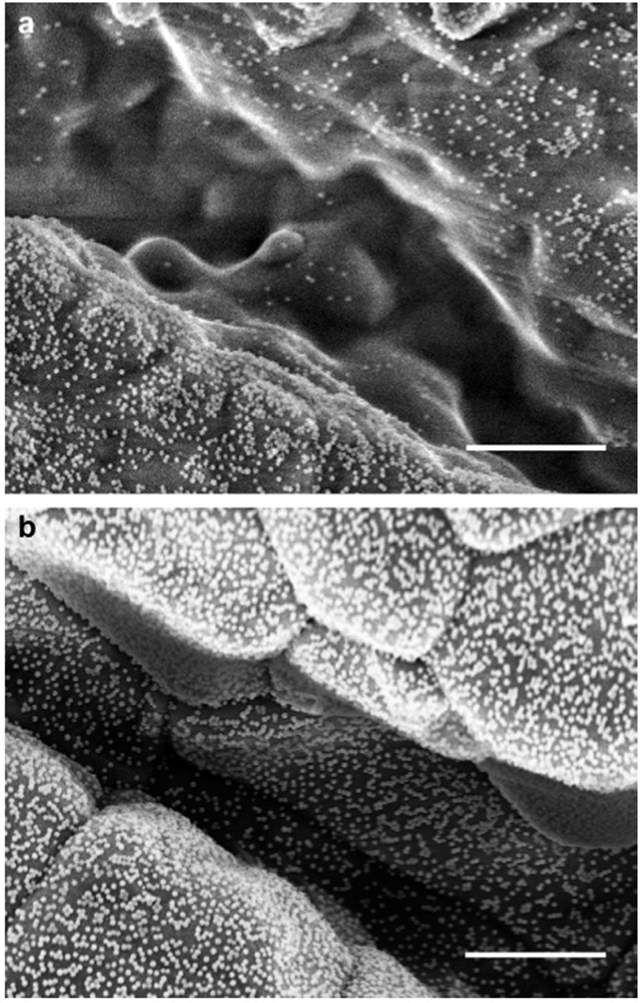
Cell Reaction
Mineralization experiments were performed and an osteopontin marker was used to quantify the mineralization of the cells. Osteopontin production steadily increased with increasing microroughness, however, an intermediate nanofeature density additionally enhanced osteopontin production (see Fig. 3).
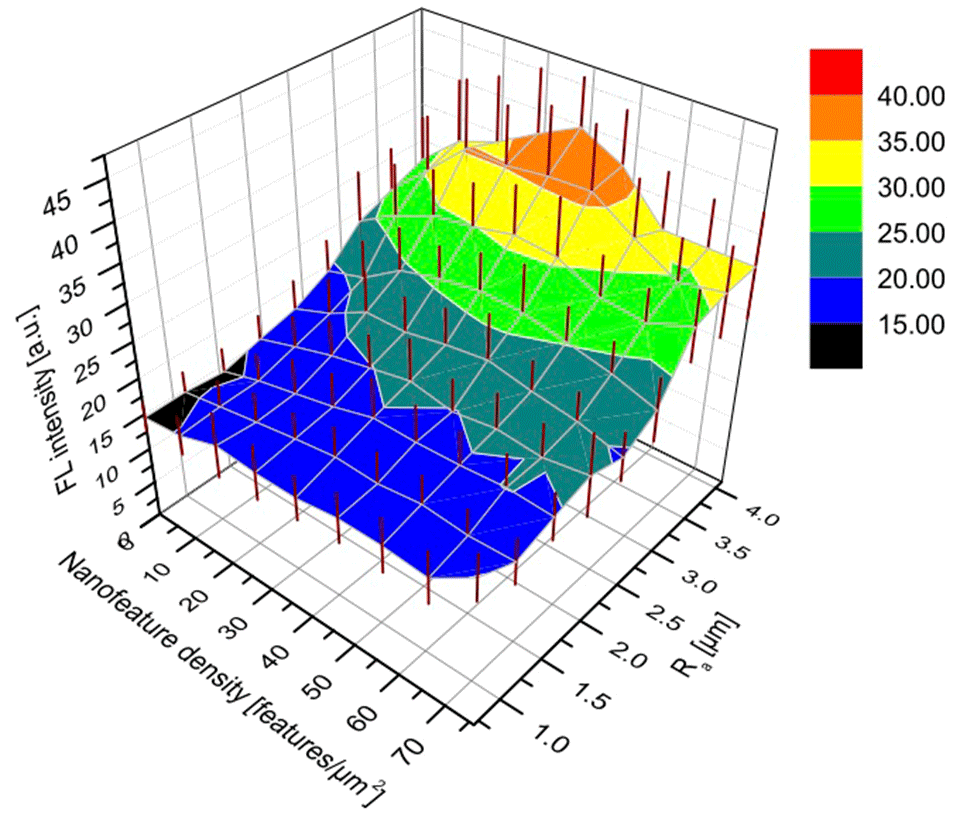
References
Zink, C.; Hall, H.; Brunette, D. M.; Spencer, N. D., external pageOrthogonal nanometer-micrometer roughness gradients probe morphological influences on cell behaviorcall_made. Biomaterials 2012, 33(32), 8055-8061.